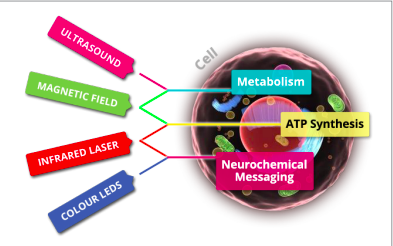
Supporting the natural self-healing ability of the body with Coherent Multi-Radiance Therapy
INTRODUCTION
I often watched as my father, a professional artist, would become inspired by the play of colours in nature. Rich and strong, or subtle and whispering, the emotional impetus echoed in me whenever I looked at his paintings. Later, when I was working as a research scientist, I studied how colours in nature carried information about the health of a tree or the mineral content of rocks. But it was only after I started my research with Coherent Multi-Radiance Therapy (coMra-Therapy) that I came to a deeper understanding of colour as a universal language, and its ability to connect not only people, but even vastly different types of intelligence: for example, human thought, emotion and even the innate “instincts” of cells that make up our body. In coMra-Therapy we use colour in concert with near infrared laser light, a magnetic field and ultrasound, and all four of these components are held together by the central idea of cooperating with the body in the healing process.
COOPERATING WITH THE CELLULAR INTELLIGENCE
In the search for medical cures during the last century we saw stunning scientific discoveries in physiology, cellular biology and biochemistry. These step by step revealed how the whole operates, based on the properties of its individual parts: body – body systems – organs – cells – molecules. This so-called reductionist approach led to many advances in allopathic medicine in the 20th century, mostly in the areas of pharmacological therapy and surgery. But now we are faced with overwhelming mountains of data on molecular, genetic and signaling processes, and this escalating complexity prevents us from understanding even the simplest forms of life [1]. Moreover, researchers who work on the cutting edge of biological sciences are well aware and acknowledge that we are hard up against the so-called “emergent” properties of cells; that is when a whole cannot be reduced to the simpler properties of its parts. We seem to have reached a stalemate: if all our hopes of health and wellbeing are pinned to multi-billion dollar research programs aimed at deciphering the complexity of the body, why do they inevitably lead to more expensive but less and less effective healthcare? Is there a viable alternative?
ADDRESSING THE NEEDS OF THE DISEASED CELLS
Imagine if we approached the body, not as a chemical/mechanical automaton, but as a highly evolved and intelligent entity that not only knows best how to maintain homeostasis, but also knows how to heal itself after an injury. Accept the concept of the “Healer Within” as at least a working hypothesis. The aim of healing then shifts from fixing “broken” individual chemical processes, to finding ways of how to help the body in its task of self-healing. This aim is as old as the arts of healing themselves, but if we place it within the context of modern science, it instructs us to focus our medical research on studying how the needs of diseased cells can be met. We will now consider this in detail.
First of all, we know that in initiating a healing sequence the cells at the site of an injury engage in “conversation” through neural and chemical cross-talk. This involves a very large number of appropriate messages delivered to different types of cells that all play roles during different stages of healing. For example, in case of a skin wound, along with pain signals from sensory nerves the resident injured cells release proinflammatory messenger
molecules that recruit immune cells to fight intruding pathogens [2]. Later, the inflammation has to stop and so then follows a tissue regeneration stage. Other types of messengers, such as Growth Factors, stimulate the proliferation, growth and maturation of young cells that will replace the damaged cells in the wound. But even the cell proliferation stage needs eventually to stop, in order to avoid excessive growth. The final stage of tissue remodeling allows functional normalization of the damaged tissue. Therefore, delivering proper messages to cells is a crucial component in any healing process. The question is how we can send such messages without disrupting the natural flow of chemical communication?
Following on from outlining the healing sequences, we need to consider the fact that the healing process requires energy. How do we feel when we get seriously sick or injured? Weakness and a desire to rest are the hallmarks of illness, because the body reroutes available energy to compensate for stress, operate the immune system and regenerate damaged cells. The energy crisis deepens even further when illness or injury decreases the ability of a cell to replenish its energy stores of the ATP molecules, the universal fuel in the body. This can happen because of a lack of oxygen or supply of nutrients, (e.g. reduced blood supply), poisoning by toxic agents or damage to the energy-producing structures (e.g. mitochondria). Therefore, energy deficit is a common denominator in practically all diseases [2; 3] and by addressing the lack of energy in cells we would certainly help the body to recover faster.
Lastly, repairing injured cells involves restoring their structure through the synthesis and degradation of cellular building blocks. The speed of these processes, jointly known as metabolism, depends on many factors, but of particular importance are temperature and the speed of molecule transfer between different sites of chemical transformation. Both these factors can be increased by providing mechanical stimulus to cells.
NON-INVASIVE STIMULATION OF THE HEALING PROCESS
We have considered several key challenges of diseased cells that can be addressed if we find therapies which avoid grossly invasive manipulation of the body’s chemistry or mechanics. In this context the uses of a magnetic field, ultrasound, colour light and near infrared lasers are of great interest. These radiances have proven their therapeutic benefits and have developed into therapeutic modalities in their own right.
Phototherapy
Phototherapy is the application of light for healing. It is a well-established therapeutic modality: in 1903 already the Nobel prize for medicine was awarded to Finsen for his work on therapy with blue light. More than a century later we know much more about physiological responses to light of certain wavelengths (colours) and the photochemical and photobiological processes that are involved. Yet we can experience quickly for ourselves the effects of phototherapy by simply observing our emotional responses to colour. For example, the colour red excites and stimulates, whereas indigo-violet calms and relaxes. Closely following our emotional state are changes in our physiological responses: such as heart rate, breathing and muscle tone. Therefore, with careful observation one can match colours to certain physiological responses and then use this knowledge for healing purposes.
Naturally, the principles of phototherapy can be best demonstrated on the healing of eye-related diseases. In the 1920s Spitler pioneered syntonic optometry: the application of colour light directly to eyes to treat visual dysfunctions, head trauma, headaches, problems with learning and behavior [4; 5]. He collated his experiences with more than 3000 patients and formulated a set of guidelines that prescribe certain colour filters to normalize (syntonize) emotional, mental and visual disorders. Red and orange colours stimulate the sympathetic nervous system (“fight or flight” response). Indigo and blue colours activate the parasympathetic nervous system (“rest and digest” response). In the mid-section between these two opposites, the yellow-green colour promotes physiological balance. Pupil and visual field testing allow objective measurements of the healing progress. Syntonic optometry has stood the test of time and today is in active use worldwide.
Remarkably, a selective response to light colour was found not to be limited to specialized sensory nerves in the eye! It can be argued that in syntonic optometry the modulation of nervous and endocrine systems happens in the thalamic and hypothalamic regulatory centers of the brain, as a result of direct visual stimulus. But non visual nerves can also perceive and distinguish between different colour stimuli. In 1947 Arvanitaki and Chalazonitis reported how light directly excites or inhibits nerve firings, depending on the wavelength of light [6; 7]. Further research in this area has led to a whole new field of nerve stimulation with light [8; 9].
But to fully appreciate colour as a universal biological language we need to turn to the works of Karu, who from the 1980s has been experimentally proving that selective responses to different wavelengths of light is a common property of cells [10-12]. She applied light of different wavelengths (blue to near infrared) to
mammalian cell cultures and demonstrated how their activity (rate of proliferation, synthesis of DNA) strongly depends on the exact wavelength (colour) of light. Moreover, and in addition to this, cells were shown to respond differently when colours were applied in sequences. For example, consecutive irradiation of blue (404 nm) and then red (633 nm) light synergetically stimulated cell response. A number of cell signaling processes were proposed to explain the observations [11; 13-16].
Low-Level Laser Therapy (LLLT)
A laser is a device that emits a highly coherent beam of light. Unlike with sunlight or light from incandescent lamps, photons of light in the laser beam travel through space in a highly synchronous and coherent way. This coherency emphasizes the energetic aspect of light. High-power lasers for cutting are very well known in surgery, but very soon after the discovery of laser technology in the 1960s experiments with noninvasive low-level lasers showed unexpected beneficial effects on wound healing [17]. Over the next four decades laboratory experiments and randomized clinical trials established LLLT as a proven therapeutic modality in a wide range of diseases: for example, neck pain [18], low back pain [19], diabetic foot wounds [20], osteoarthritic knee pain [21], amblyopia or “lazy eye” [22] to name but a few. “The New Laser Therapy Handbook” published in 2010 by Tunér and Hode lists 130 randomized clinical trials, providing a level of evidence equal to or exceeding that of many drugs on the market today [23].
For anyone who is accustomed to the narrow scope of actions of drugs the therapeutic universality of lasers immediately raises questions about how it is possible to treat vastly different conditions with the same therapy? Yet experiments have clearly demonstrated lasers’ normalizing effects on inflammation [24-26], regeneration of skin, muscle, bone and nervous tissue [27-31], growth of new blood vessels in wounds [32], rescue of neurons inactivated by toxins [33; 34], and stimulation of immune cells [35]. The search for a mechanism that could explain such different manifestations of LLLT led to the discovery that LLLT stimulates synthesis of the universal fuel of cells – the ATP molecule [36-39]. Near doubling in concentrations of ATP were detected after irradiation with near Infrared and red light in animal cells cultivated in vitro (in test tube). Later, in vivo (in living organism) experiments confirmed that near Infrared irradiation restored cortical ATP content after embolic stroke [40] and traumatic brain injury [41]. Providing cells with extra energy allows for a natural resolution of the disease, regardless of its type.
Magnetotherapy and Magneto-Infrared-Laser Therapy (MIL-Therapy)
Therapies using naturally-occurring magnetic materials have been known for centuries [42]. Modern day clinical evidence has confirmed the efficacy of magnetotherapy across areas as diverse as bone unification [43; 44], edema of soft tissues and pain relief [45-47].
In an interesting turn of events, similarities between some of the clinical effects of LLLT and magnetotherapy led to experiments with their combined application. In the late 1970s Russian scientists proposed the idea of Magneto-Infrared-Laser Therapy, (MIL-Therapy), and by the 1990s MIL-Therapy devices were introduced to clinical practice [48]. A combination of the two low-level radiances showed synergetic healing effects that exceeded those of the individual radiances. Over the past two decades the technology has proved to be so successful that it is now used in leading Russian medical institutes to treat more than 200 medical conditions across such fields as traumatology, infectious diseases, urology, dentistry, neurology, endocrinology, and many others [49-53]. Unfortunately, the clinical results are mostly published in Russian (see peer reviewed journal “Laser Medicine” at http://elibrary.ru/issues.asp?id=8801 and the thematic collection of papers cited above) and they are therefore not well known elsewhere.
How magnetotherapy works at a cellular level and especially the nature of its synergy with low-level lasers remained uncertain until very recently. The latest discoveries in isotope chemistry, however, have shown clearly that the key process of energy metabolism (ATP synthesis) can be stimulated by a magnetic field [54-56]. Therefore, we can see that it is possible to use both low-level lasers and a magnetic field to help cells suffering from energy deficit. And here we need to note that practically all types of cells can benefit from such stimulus; in humans, as well as in animals.
Ultrasound Therapy
Ultrasound is an acoustic pressure wave (>20kHz) that passes through tissue as a train of compression and expansion zones creating vibration [57]. Therapeutic use of ultrasound has existed since 1927 [58] and in clinical practice ultrasound has been used for decades for accelerating bone repair [59-61] and wound healing [62; 63]. Experiments with low intensity ultrasound have shown effects similar to phototherapy, LLLT and magnetotherapy with respect to proliferation of cells [64], growth of new blood vessels after injury [65] and enhancement of blood circulation [66]. Many studies have compared ultrasound with LLLT in terms of the overall healing [67-69], but in the context of our discussion of coMra-Therapy we need to identify differences for, obviously, ultrasound acts very differently to low-level laser.
Ultrasound stimulates metabolism in the body through increases in molecular transport and temperature, thus speeding up processes of protein synthesis/degradation that are crucial for restoring damaged tissues [70; 71]. Specifically it has been suggested that ultrasound accelerates enzymatic activity [72]. In the body most chemical reactions are assisted by enzymes, the protein catalysts that bring together input molecules, allowing them to undergo chemical change and then the product is released from the enzyme. Ultrasound can increase the speed of delivery of substrate to an enzyme’s active site and also the subsequent dislodging of the product. For example, experiments of blood clot dissolution with ultrasound showed that the speed of noninvasive enzymatic degradation of the clot can increase from 2 to 5.5 times in the presence of ultrasound [73-75]. Besides the purely mechanical stimulation of enzymatic activity, ultrasonic energy is eventually absorbed in tissue and this very slight heating also increases the rate of biochemical reactions [71; 76].
PUTTING IT ALL TOGETHER
We have considered experimental and clinical evidence concerning the four therapeutic radiances. When applied at optimum doses, including wavelengths (frequencies), with modulation/pulsing and other parameters, all of these radiances have been shown to be therapeutically effective. The absence of negative side effects is typical with these low-level therapies. And all of these therapies to different degrees have shown beneficial effects on cell proliferation, inflammation, pain relief and immunity to disease, in cases with very different underlying pathologies. Realization of these facts has led to a large number of comparative experiments, clinical trials and systematic reviews indicating the best modality for a certain condition [21; 77-79]. But could these radiances be grouped together to support the healing process?
Obviously the four radiances operate according to different physical/chemical bases. Incoherent and coherent light, magnetism and ultrasound are very different types of radiant energy. But in the analysis I presented above, I showed how the four radiances play their specialized roles in three crucial aspects underlying the natural way of resolving a disease at a cellular level (Figure 1).
Note that these vital and tightly connected aspects do not compete against each other! Instead, if stimulated coherently they result in the emergence of a synergetic healing effect: the whole is greater than the sum of its parts. The opposite is equally true: if we stimulate only some of these aspects, the overall healing process will still benefit, but only up to the limits imposed by the slowest process. These principles of holism are the cornerstone of Coherent Multi-Radiance Therapy.
FEATURES OF coMra-THERAPY
The concept of coMra-Therapy, which included the blueprint for combining the four therapeutic radiances, and the main parameters of operation, came about following an intuitive discovery, specifically, a dream. Based on the information in the dream, we at Radiant Life Technologies continued the practical development of coMra Therapy and the Delta Laser by assimilating the more esoteric principles of colour and intuition and combining these with principles of medical science, together with a knowledge of healing, and clinical experiences of the individual radiances, including those of MIL-Therapy.
Near Infrared Laser and Magnetic Field
Near infrared laser is the central component of coMra-Therapy. It provides energetic stimulus for the whole healing process. A selection of two laser diodes are used in coMra-Therapy (905 or 980nm) at 10 mW radiant power. (The 905nm laser is available to practitioners on special order). Light at both these wavelengths has superior penetration abilities in tissue, since at longer wavelengths light is more absorbed by water and at shorter wavelengths light is absorbed by skin pigments and hemoglobin [80]. With this laser light direct stimulation of ATP synthesis is possible in diseased tissues, even deep in the body (up to 5 cm [81]).
The strength of the static magnetic field in coMra-Therapy is 30 mT, although this varies due to spatial gradient and shape of the magnetic field. The magnetic field strongly enhances the effects of the laser, making the commonly-used higher doses of irradiation in LLLT unnecessary. Friedmann et al experimentally demonstrated that a weak magnetic field increased the effect of the infrared laser by an order of magnitude [82].
Sequenced Colour LEDs
The light from colour Light Emitting Diodes (LEDs) is fully absorbed by the skin, and provides a healing message to skin-embedded sensory nerves. Since our skin represents the body’s largest organ, possessing vital immune and endocrine functions [83; 84], LED irradiation of skin also initiates both local and systemic responses to disease, through chemical messengers (cytokines, hormones) [85].
The three sets of LEDs (650nm, 420nm, 570nm) are modulated in one of two specific sequences in an on/ off pattern. One pattern is the regenerative message, and follows the Red –> Indigo-violet –> Yellow/Green sequence. It represents what esotericists term Life Coming Into Manifestation, a pattern that is responsible for evolution or generation. This pattern also corresponds to the natural progression in the wound healing process discussed above: Inflammation (Red) –> Cell Proliferation (Indigo-Violet) –> Wound Remodeling (Yellow/ Green). The other pattern is the rejuvenative message and follows the colour sequence Red –> Yellow/Green –> Indigo-violet. It corresponds to what esotericists call Life Within Manifestation, a pattern that is responsible for enhancing the existing structures, including cells, within the body. This second pattern is rejuvenative in nature, as it promotes enhancement of existing tissue. This makes it suited to, for example, cosmetology. Consequently this pattern is incorporated within one of the more specialised embodiments of coMra-Therapy.
Ultrasound
Ultrasound in coMra-Therapy is used at the frequency of 40 kHz. This has greater penetration than megahertz based therapeutic frequencies, and does not cause excessive heating [74]. The ultrasound emitters are placed at a 9 mm distance from the skin to provide a very gentle stimulation of enzymatic metabolism. Only in the embodiment for skin rejuvenation are the emitters placed at skin level for additional stimulation.
Geometry and modulation
The geometry, as well as the temporal modulation of the radiances are crucial aspects for achieving overall coherence in coMra-Therapy. Each radiance emitter is therefore positioned specifically according to the role of the particular radiance, and to achieve simultaneous irradiation of the same volume of tissue (Figure 2). Ultrasound emitters and LEDs/magnets are placed radially from the central laser diode.
As a result of experiments conducted with MIL-Therapy it was shown that on/off modulation of the laser diode enables a maximum effect to be achieved in terms of the effective penetration or depth. Recent experiments have confirmed this phenomenon [86]. Modulating at 5Hz the laser effect achieves a maximum effective penetration, reaching inner organs and bone. At 1000Hz it penetrates epidermis and dermis only. At 50Hz the effective penetration is moderate, (through subcutaneous layers of tissue and superficial muscles) and with the Variable setting the laser is set to sweep gradually through the range of modulation frequencies. LED sequencing is linked to the modulation of the laser diode, but at lower harmonic frequencies.
Application of coMra-Therapy
It is not possible within the space of this article to describe either the principles of application of coMra-Therapy to the body or the clinical experiences of coMra-Therapy. Our aim is to complement our existing collection of studies with an ever-increasing number of reports and clinical trials with coMra-Therapy. I would nonetheless like to outline some pertinent conclusions arising from the combination of radiances that show coMra-Therapy to be such a breakthrough in the evolution of noninvasive medicine.
The emergent synergetic effects of the four radiances make it possible to use them at lower intensities than in monotherapies. For example, a direct comparison of the 980 nm near infrared laser used in coMra-Therapy at 10 mW with a similar monotherapy (LLLT), would reveal the healing potential of the monotherapy, but would not show the healing efficacy of coMra-Therapy.
Another consequence of the combination of radiances is that the recommended doses of coMra-Therapy (times of application) are much lower than in monotherapies. At the same time, there is also no risk of any overdose of coMra-Therapy. LLLT practitioners are well aware of the need for an exact dosage, because of the strong reductions in therapeutic effects in the event of overdosing [87-89]. But for a coMra-Therapy user it is not uncommon to treat a headache after a long day in the office, a newly-appeared back pain, chronic pain in the knees and an allergy during one evening, without any negative effects.
After learning the basics of using coMra-Therapy, anyone can safely design the best treatment plans him or herself in the case of home user. In the case of a health practitioner he or she can use specific medical knowledge and expertise to fine-tune treatment plans for serious/critical patients. A comprehensive User Guide has been developed with illustrated treatment protocols covering over 200 medical conditions. However the principles of treatment are fairly simple. coMra-Therapy is applied primarily directly over the site of injury and over the organs, blood, lymph, endocrine and nervous systems that are involved in the pathological process. coMra-Therapy also can be used to deliver a healing stimulus to reflexology pressure points and acupuncture points.
CONCLUDING REMARKS
The responsibility for our health at the end of the day rests in our own hands. To give the body love and care is again a choice that every individual can make. Therefore it is the direct responsibility of science and technology to make these choices possible for everyone. It is my greatest hope that after reading this article you will be able to see that medical science and the arts of healing blend into each other casually and naturally, once we approach the body with respect and knowledge.
If you feel that you would like to join us to add your skills and talents to ours, by working with coMra-Therapy and bringing its approaches to benefit your life or the life of your community, please contact us at info@radiant-life-technologies.com. RLT is also open to receiving proposals for basic research and clinical trials.
ACKNOWLEDGEMENTS
I am immensely grateful to the late Théun Mares, the founder of Radiant Life Technologies, who shared and inspired me with his vision of the science of coMra-Therapy. And to my dear friends at Radiant Life Technologies: thank-you for your warmth, your unwavering support and for sharing our journey!
REFERENCES
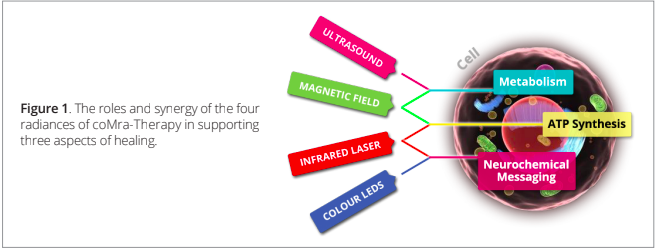

- Alberts, B. 2012. The End of “Small Science”? Science no. 337 (6102):1583. doi: 10.1126/science.1230529. 2. Kumar, V., et al. 2007. Robbins basic pathology. 8 ed. Philadelphia, PA: Saunders/Elsevier.
- Seppet, E., et al. 2009. Mitochondria and energetic depression in cell pathophysiology. International Journal of Molecular Sciences no. 10 (5):2252-303. doi: 10.3390/ijms10052252.
- Spitler, H.R. 1941. The Syntonic Principle. Eaton, Ohio: College of Syntonic Optometry. Reprint, 1990.
- Gottlieb, R.L., et al. 2010. Syntonic phototherapy. Photomedicine and Laser Surgery no. 28 (4):449-452. doi: 10.1089/pho.2010.9933.
- Arvanitaki, A., et al. 1961. “Excitatiory and inhibitory processes initiated by light and infra-red radiations in single identifiable nerve cells.” In Nervous inhibition, edited by E. Florey, 194-231. Oxford: Pergamon. doi: 10.5962/bhl.title.7235
- Arvanitaki, A., et al. 1947. Reactiones bioelectriques a la photoactivation des cytochromes. Arch. Sci. Physiol. no. 1:385-405.
- Richter, C.P., et al. 2011. Neural stimulation with optical radiation. Laser & Photonics Reviews no. 5 (1):68- 80. doi: 10.1002/lpor.200900044.
- Fork, R.L. 1971. Laser Stimulation of Nerve Cells in Aplysia. Science no. 171 (3974):907-908. doi: 10.1126/science.171.3974.907.
- Karu, T., et al. 1985. Biostimulation of HeLa cells by low-intensity visible light. Il Nuovo Cimento D no. 5 (6):483-496. doi: 10.1007/bf02452546.
- Karu, T., et al. 1994. Two different mechanisms of low-intensity laser photobiological effects on Escherichia coli. Journal of Photochemistry and Photobiology B: Biology no. 24 (3):155-61. doi: 10.1016/1011-1344(94)07016-4.
- Karu, T.I., et al. 2005. Exact Action Spectra for Cellular Responses Relevant to Phototherapy. Photomedicine and Laser Surgery no. 23 (4):355-361. doi: 10.1089/pho.2005.23.355.
- Karu, T.I. 1989. Photobiology of low-power laser effects. Health Physics no. 56 (5):691-704.
- Karu, T.I., et al. 2004. A novel mitochondrial signaling pathway activated by visible-to-near infrared radiation. Photochemistry and Photobiology no. 80 (2):366-372. doi: 10.1111/j.1751-1097.2004.tb00097.x.
- Safavi, S., et al. 2008. Effects of low-level He–Ne laser irradiation on the gene expression of IL-1β, TNF-α, IFN-γ, TGF-β, bFGF, and PDGF in rat’s gingiva. Lasers in Medical Science no. 23 (3):331-335. doi: 10.1007/s10103-007-0491-5.
- Anders, J.J., et al. 2008. Light supports neurite outgrowth of human neural progenitor cells in vitro: the role of P2Y receptors. IEEE Journal of Selected Topics in Quantum Electronics no. 14 (1):118-125. doi: 10.1109/JSTQE.2008.916181.
- Mester, E., et al. 1971. Effect of laser rays on wound healing. The American Journal of Surgery no. 122 (4):532-535. doi: 10.1016/0002-9610(71)90482-x.
- Chow, R.T., et al. 2009. Efficacy of low-level laser therapy in the management of neck pain: a systematic review and meta-analysis of randomised placebo or active-treatment controlled trials. The Lancet no. 374 (9705):1897-1908. doi: 10.1016/S0140-6736(09)61522-1.
- Konstantinovic, L.M., et al. 2010. Acute low back pain with radiculopathy: a double-blind, randomized, placebo-controlled study. Photomedicine and Laser Surgery no. 28 (4):553-60. doi: 10.1089/pho.2009.2576.
- Kaviani, A., et al. 2011. A randomized clinical trial on the effect of low-level laser therapy on chronic diabetic foot wound healing: a preliminary report. Photomedicine and Laser Surgery no. 29 (2):109-14. doi: 10.1089/pho.2009.2680.
- Bjordal, J., et al. 2007. Short-term efficacy of physical interventions in osteoarthritic knee pain. A systematic review and meta-analysis of randomised placebo-controlled trials. BMC Musculoskeletal Disorders no. 8 (1):51. doi: 10.1186/1471-2474-8-51.
- Ivandic, B., et al. 2012. Low-Level Laser Therapy Improves Visual Acuity in Adolescent and Adult Patients with Amblyopia. Photomedicine and Laser Surgery no. 30 (3):167-171. doi: 10.1089/pho.2011.3089.
- Tunér, J., et al. 2010. The new laser therapy handbook. Gröngesberg: Prima Books.
- Bjordal, J.M., et al. 2010. The anti-inflammatory mechanism of low level laser therapy and its relevance for clinical use in physiotherapy. Physical Therapy Reviews no. 15 (4):286-293. doi: 10.1179/1743288x10y.0000000001.
- Yamaura, M., et al. 2009. Low level light effects on inflammatory cytokine production by rheumatoid arthritis synoviocytes. Lasers in Surgery and Medicine no. 41 (4):282-290. doi: 10.1002/lsm.20766.
- Aimbire, F., et al. 2006. Low-level laser therapy induces dose-dependent reduction of TNFα levels in acute inflammation. Photomedicine and Laser Surgery no. 24 (1):33-37. doi: 10.1089/pho.2006.24.33.
- Hawkins, D., et al. 2006. Effect of multiple exposures of low-level laser therapy on the cellular responses of wounded human skin fibroblasts. Photomedicine and Laser Surgery no. 24 (6):705-714. doi: 10.1089/pho.2006.24.705.
- de Almeida, P., et al. 2011. Low-level Laser Therapy Improves Skeletal Muscle Performance, Decreases Skeletal Muscle Damage and Modulates mRNA Expression of COX-1 and COX-2 in a Dose-dependent Manner. Photochemistry and Photobiology no. 87 (5):1159-1163. doi: 10.1111/j.1751-1097.2011.00968.x.
- Ferraresi, C., et al. 2011. Effects of low level laser therapy (808 nm) on physical strength training in humans. Lasers in Medical Science no. 26 (3):349-358. doi: 10.1007/s10103-010-0855-0.
- Grassi, F.R., et al. 2011. Effect of low-level laser irradiation on osteoblast proliferation and bone formation. Journal of biological regulators and homeostatic agents no. 25 (4):603-14.
- Rochkind, S., et al. 2009. Phototherapy in peripheral nerve injury: effects on muscle preservation & nerve regeneration. International Review of Neurobiology no. 87:445-64. doi: 10.1016/S0074-7742(09)87025-6.
- Sousa, A., et al. 2012. Laser and LED phototherapies on angiogenesis. Lasers in Medical Science. doi: 10.1007/s10103-012-1187-z.
- Wong-Riley, M.T.T., et al. 2005. Photobiomodulation Directly Benefits Primary Neurons Functionally Inactivated by Toxins. Journal of Biological Chemistry no. 280 (6):4761-4771. doi: 10.1074/jbc.M409650200.
- Liang, H.L., et al. 2006. Photobiomodulation partially rescues visual cortical neurons from cyanide induced apoptosis. Neuroscience no. 139 (2):639-649. doi: 10.1016/j.neuroscience.2005.12.047.
- Morgan, M.C., et al. 2009. The effect of phototherapy on neutrophils. International Immunopharmacology no. 9 (4):383-388. doi: 10.1016/j.intimp.2009.02.001.
- Passarella, S., et al. 1984. Increase of proton electrochemical potential and ATP synthesis in rat liver mitochondria irradiated in vitro by helium-neon laser. FEBS Letters no. 175 (1):95-99. doi: 10.1016/0014-5793(84)80577-3.
- Karu, T., et al. 1995. Irradiation with HeNe laser increases ATP level in cells cultivated in vitro. Journal of Photochemistry and Photobiology B: Biology no. 27 (3):219-223. doi: 10.1016/1011-1344(94)07078-3.
- Pastore, D., et al. 1996. Stimulation of ATP synthesis via oxidative phosphorylation in wheat mitochondria irradiated with Helium-Neon laser. IUBMB Life no. 39 (1):149-157. doi: 10.1080/15216549600201151.
- Oron, U., et al. 2007. Ga-As (808 nm) laser irradiation enhances ATP production in human neuronal cells in culture. Photomedicine and Laser Surgery no. 25 (3):180-182. doi: doi:10.1089/pho.2007.2064.
- Lapchak, P.A., et al. 2010. Transcranial near infrared laser treatment (NILT) increases cortical adenosine-5’-triphosphate (ATP) content following embolic strokes in rabbits. Brain Research no. 1306:100-105. doi: 10.1016/j.brainres.2009.10.022.
- Ando, T., et al. 2011. Comparison of Therapeutic Effects between Pulsed and Continuous Wave 810- nm Wavelength Laser Irradiation for Traumatic Brain Injury in Mice. PLoS One no. 6 (10):e26212. doi: 10.1371/journal.pone.0026212.
- Markov, M.S. 2007. Magnetic field therapy: a review. Electromagnetic Biology and Medicine no. 26 (1):1-23. doi: 10.1080/15368370600925342.
- Bassett, C.A.L., et al. 1974. Acceleration of fracture repair by electromagnetic fields. A surgically noninvasive method. Annals of the New York Academy of Sciences no. 238 (1):242-262. doi: 10.1111/j.1749-6632.1974.tb26794.x.
- Mooney, V. 1990. A randomized double-blind prospective study of the efficacy of pulsed electromagnetic fields for interbody lumbar fusions. Spine no. 15 (7):708-712.
- Weintraub, M.I., et al. 2003. Static magnetic field therapy for symptomatic diabetic neuropathy: a randomized, double-blind, placebo-controlled trial. Archives of Physical Medicine and Rehabilitation no. 84 (5):736-746. doi: 10.1016/S0003-9993(03)00106-0.
- Brown, C.S., et al. 2002. Efficacy of static magnetic field therapy in chronic pelvic pain: a double-blind pilot study. American Journal of Obstetrics and Gynecology no. 187 (6):1581-1587.
- Eccles, N.K. 2005. A critical review of randomized controlled trials of static magnets for pain relief. Journal of Alternative and Complementary Medicine no. 11 (3):495-509. doi: 10.1089/acm.2005.11.495.
- Illarionov, V.E. 2009. Magnetotherapy. Moscow: Libricom. [in Russian].
- Ivanchenko, L.P., et al. 2009. Laser therapy in urology. Moscow - Tver: Triada Publishing House. [in Russian].
- Moskvin, S.V., et al. 2007. Therapy with matrix impulse lasers of red spectrum. Moscow - Tver: Triada Publishing House. [in Russian].
- Pavlov, S.E. 2008. Laser therapy with matrix magneto-infrared laser devices. Moscow: Space Device Corporation. [in Russian].
- Zhilin, J.N. 2002. Quantum therapy for phthisiology and pulmonology. Moscow: Association of quantum medicine. [in Russian].
- Potemkin, L.A. 2001. Medical science and quantum medicine for elite sportsmen. Moscow: Space Device Corporation. [in Russian].
- Buchachenko, A.L., et al. 2005. Magnetic isotope effect of magnesium in phosphoglycerate kinase phosphorylation. Proceedings of the National Academy of Sciences of the United States of America no. 102 (31):10793-10796. doi: 10.1073/pnas.0504876102.
- Buchachenko, A.L., et al. 2008. Magnetic field affects enzymatic ATP synthesis. Journal of the American Chemical Society no. 130 (39):12868-12869. doi: 10.1021/ja804819k.
- Buchachenko, A.L., et al. 2012. Chemistry of Enzymatic ATP Synthesis: An Insight through the Isotope Window. Chemical Reviews no. 112 (4):2042-2058. doi: 10.1021/cr200142a.
- Leighton, T.G. 2007. What is ultrasound? Progress in Biophysics and Molecular Biology no. 93 (1–3):3-83. doi: 10.1016/j.pbiomolbio.2006.07.026.
- Wood, R.W., et al. 1927. The physical and biological effects of high frequency sound waves of great intensity. Phil. Mag no. 4:417–436.
- Khan, Y., et al. 2008. Fracture repair with ultrasound: clinical and cell-based evaluation. The Journal of Bone and Joint Surgery no. 90:138-44. doi: 10.2106/JBJS.G.01218.
- Pounder, N.M., et al. 2008. Low intensity pulsed ultrasound for fracture healing: a review of the clinical evidence and the associated biological mechanism of action. Ultrasonics no. 48 (4):330-338. doi: 10.1016/j.ultras.2008.02.005.
- Claes, L., et al. 2007. The enhancement of bone regeneration by ultrasound. Progress in Biophysics and Molecular Biology no. 93 (1-3):384-398. doi: 10.1016/j.pbiomolbio.2006.07.021.
- Kavros, S.J., et al. 2007. Treatment of ischemic wounds with noncontact, low-frequency ultrasound: the Mayo clinic experience, 2004-2006. Advances in Skin & Wound Care no. 20 (4):221-226. doi: 10.1097/01.ASW.0000266660.88900.38.
- Weichenthal, M., et al. 1997. Low-frequency ultrasound treatment of chronic venous ulcers. Wound Repair and Regeneration no. 5 (1):18-22. doi: 10.1046/j.1524-475X.1997.50107.x.
- Tsuang, Y.-H., et al. 2011. Effects of Low Intensity Pulsed Ultrasound on Rat Schwann Cells Metabolism. Artificial Organs no. 35 (4):373-383. doi: 10.1111/j.1525-1594.2010.01086.x.
- Young, S.R., et al. 1990. The effect of therapeutic ultrasound on angiogenesis. Ultrasound in Medicine & Biology no. 16 (3):261-269. doi: 10.1016/0301-5629(90)90005-w.
- Fischell, T.A., et al. 1991. Ultrasonic energy. Effects on vascular function and integrity. Circulation no. 84 (4):1783-95. doi: 10.1161/01.cir.84.4.1783.
- Rennó, A.C.M., et al. 2011. Comparative Effects of Low-Intensity Pulsed Ultrasound and Low-Level Laser Therapy on Injured Skeletal Muscle. Photomedicine and Laser Surgery no. 29 (1):5-10. doi: 10.1089/pho.2009.2715.
- Fávaro-Pípi, E., et al. 2010. Comparative study of the effects of low-intensity pulsed ultrasound and low-level laser therapy on bone defects in tibias of rats. Lasers in Medical Science no. 25 (5):727-732. doi: 10.1007/s10103-010-0772-2.
- Bakhtiary, A.H., et al. 2004. Ultrasound and laser therapy in the treatment of carpal tunnel syndrome. The Australian Journal of Physiotherapy no. 50 (3):147-51.
- ter Haar, G. 2007. Therapeutic applications of ultrasound. Progress in Biophysics and Molecular Biology no. 93 (1–3):111-129. doi: 10.1016/j.pbiomolbio.2006.07.005.
- Baker, K.G., et al. 2001. A review of therapeutic ultrasound: biophysical effects. Physical Therapy no. 81 (7):1351-1358.
- Johns, L.D. 2002. Nonthermal effects of therapeutic ultrasound: the frequency resonance hypothesis. Journal of Athletic Training no. 37 (3):293-9.
- Blinc, A., et al. 1993. Characterization of ultrasound-potentiated fibrinolysis in vitro. Blood no. 81 (10):2636-43.
- Suchkova, V., et al. 1998. Enhancement of fibrinolysis with 40-kHz ultrasound. Circulation no. 98 (10):1030-1035. doi: 10.1161/01.cir.98.10.1030.
- Akiyama, M., et al. 1998. Low-frequency ultrasound penetrates the cranium and enhances thrombolysis in vitro. Neurosurgery no. 43 (4):828-832.
- Chan, A.K., et al. 1998. Temperature changes in human patellar tendon in response to therapeutic ultrasound. Journal of Athletic Training no. 33 (2):130-5.
- Laakso, E.L., et al. 2010. Electrophysical agents (EPAs) for symptom control in cancer care - what is the evidence? Physical Therapy Reviews no. 15 (4):334-343. doi: 10.1179/174328810x12786297204954.
- Kocyigit, I.D., et al. 2012. A Comparison of the Low-Level Laser Versus Low Intensity Pulsed Ultrasound on New Bone Formed Through Distraction Osteogenesis. Photomedicine and Laser Surgery no. 30 (8):1-6. doi: 10.1089/pho.2012.3263.
- Kwan, R.L.C., et al. 2012. Electrophysical therapy for managing diabetic foot ulcers: a systematic review. International Wound Journal. doi: 10.1111/j.1742-481X.2012.01085.x.
- Chung, H., et al. 2012. The nuts and bolts of low-level laser (Light) therapy. Ann Biomed Eng Annals of Biomedical Engineering no. 40 (2):516-533. doi: 10.1007/s10439-011-0454-7.
- Moskvin, S.V., et al. 2007. Laser colour therapy. Moscow - Tver: Triada Publishing House. [in Russian].
- Friedmann, H., et al. 2009. Combined magnetic and pulsed laser fields produce synergistic acceleration of cellular electron transfer. Laser Therapy no. 18 (3):137-141. doi: 10.5978/islsm.18.137.
- Slominski, A., et al. 2000. Neuroendocrinology of the skin. Endocrine Reviews no. 21 (5):457-487. doi: 10.1210/er.21.5.457.
- Bos, J.D. 2005. Skin immune system: cutaneous immunology and clinical immunodermatology. Boca Raton: CRC Press.
- Dyson, M. 2009. How photons modulate wound healing via the immune system. Paper read at Biophotonics and Immune Responses IV, at San Jose, CA, USA. doi: 10.1117/12.808291.
- Joensen, J., et al. 2012. Skin Penetration Time-Profiles for Continuous 810 nm and Superpulsed 904 nm Lasers in a Rat Model. Photomedicine and Laser Surgery no. 30 (12):688-94. doi: 10.1089/pho.2012.3306.
- Huang, Y.-Y., et al. 2011. Biphasic Dose Response in Low Level Light Therapy – An Update. Dose-Response no. 1 (1):1-17. doi: 10.2203/dose-response.11-009.Hamblin.
- Jenkins, P.A., et al. 2011. How to Report Low-Level Laser Therapy (LLLT)/Photomedicine Dose and Beam Parameters in Clinical and Laboratory Studies. Photomedicine and Laser Surgery no. 29 (12):785- 787. doi: 10.1089/pho.2011.9895.
- Huang, Y.-Y., et al. 2009. Biphasic dose response in low level light therapy. Dose-Response no. 7 (4):358- 383. doi: 10.2203/dose-response.09-027.Hamblin.